Research

© AG Quantum
Ultracold Fermions in optical lattices

© AG Quantum
Simulation of the BEC-BCS Crossover with Lithium

© AG Quantum
Ion lab

© AG Quantum
Strontium Tweezer Experiment
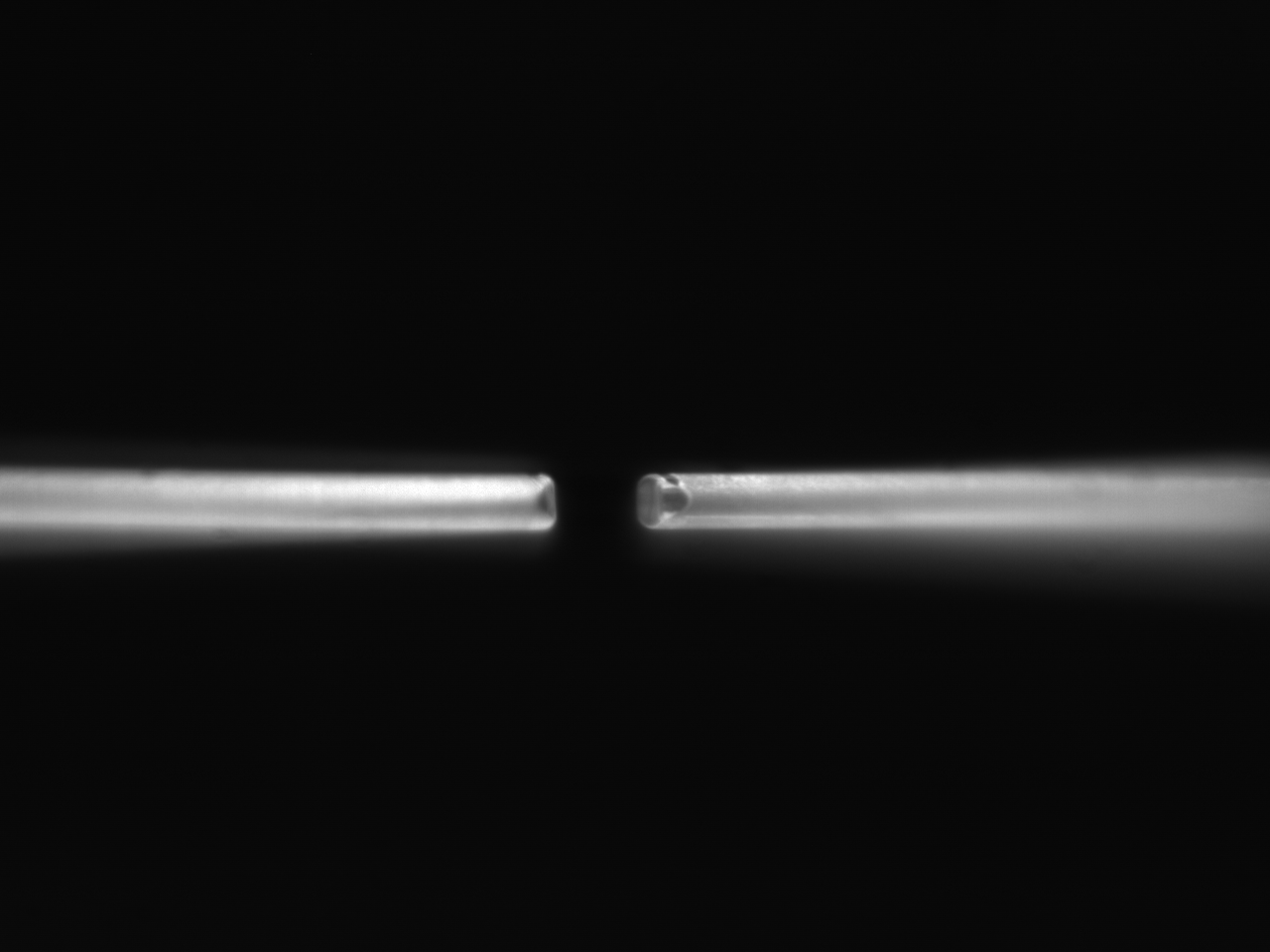
© AG Quantum
Miniature optical resonators

© AG Quantum
2D Materials
Scientific Instrumentation

© AG Quantum
Apparatus for generating and studying cold atoms
Funded by Deutsche Forschungsgemeinschaft

© AG Quantum
Laser system for high-resolution spectroscopy of atomic quantum gases
Funded by Deutsche Forschungsgemeinschaft